Chapter 6: Igneous Rocks
Classification, Mapping, and Volcanic Hazards
Learning Objectives
The goals of this chapter are to:
- Characterize and classify igneous rocks
- Analyze volcanic frequency and hazards
- Determine volcanic stratigraphic relationships
6.1 Igneous Rocks and Earth’s Layers
The upper layers (mantle and crust) of the Earth are mainly composed of igneous rocks, although sedimentary rocks are the most abundant on the surface. You probably have learned that these layers formed early in its history through the process of chemical differentiation, when denser elements sank towards the center of the Earth and less dense elements floated toward the surface. This led to density differences between the layers as well as chemical differences.
Exercise 6.1 – Determining the Density of Earth’s Layers
Your instructor has presented you with three examples of Earth’s layers: oceanic crust, continental crust, and the upper mantle.
- Describe the differences between the three rocks.
- Measure the density of each sample as you did at the end of Chapter 1. Weigh each sample and record the mass, then record how much water is displaced when you place it in a graduated cylinder. Fill out table 6.1.
Table 6.1 – Density calculation Rock Mass (g) Volume (cm3) Density (g/cm3) - How do your measured densities compare with your observations in a?
- What do you think causes the varying densities of these rocks?
- On Figure 6.1, show where these rocks could come from.
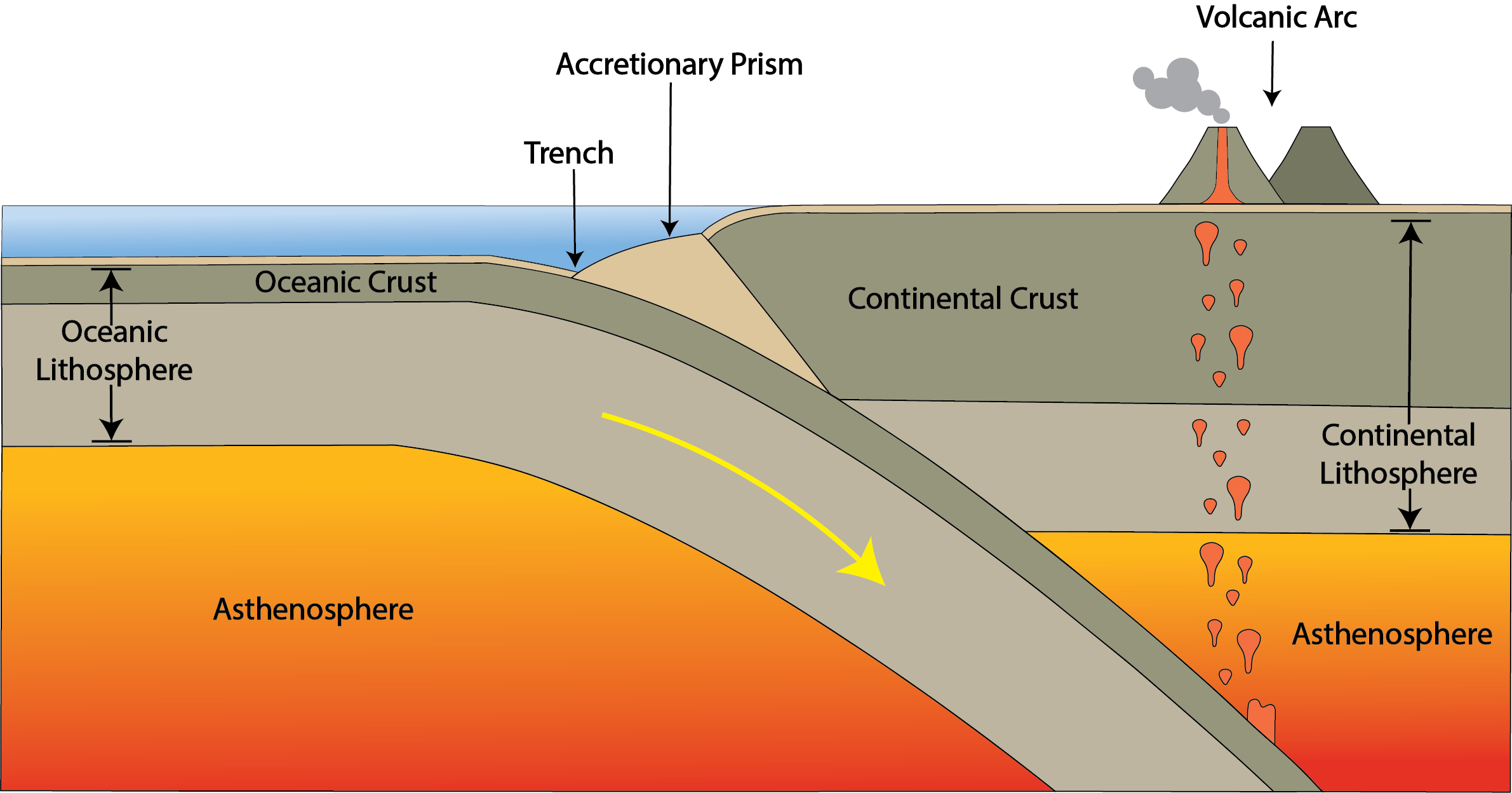
6.2 Classifying Igneous Rocks
The classification of igneous rocks is based on chemistry and texture, which means it’s based on the minerals present and how large the mineral grains are (Figures 6.2 to 6.5). The magma’s chemistry and temperature determine which minerals will form. There are eight common minerals in igneous rocks (Figure 6.2), which can be separated into four different igneous compositions: ultramafic, mafic, intermediate, and felsic.
The size of the mineral grains is chiefly determined by how quickly the magma cools. Slow cooling rates (thousands to half a million years) make larger mineral grains, creating intrusive (plutonic) igneous rocks. Fast cooling rates (instantaneous to hundreds of years) produce smaller grains, creating extrusive (volcanic) igneous rocks. The distinction between fine and coarse grains is whether you can identify the minerals without magnification. This is important because two different rocks can have the same composition, but different textures (small grains vs. large grains). For example, diorite (large grains, intrusive igneous rock) and andesite (small grains, extrusive igneous rock) have the same minerals but different textures.
The common textures of igneous rocks are:
- Coarse (phaneritic) – grains are large enough to identify without magnification, indicative of slow cooling; intrusive rocks
- Fine (aphanitic) – grains are too small to identify without magnification, indicative of fast cooling; extrusive rocks
- Porphyritic – grains are a mixture of coarse and fine grains, indicative of two cooling rates, slow at first (intrusive) and then fast (extrusive)
- Glassy – very smooth texture with no minerals, cooled so quickly that minerals did not have time to form, commonly has conchoidal fracture; extrusive rocks
- Vesicular – rough, porous texture, cooled quickly, vesicles (holes) created from abundant gas in the lava; extrusive rocks
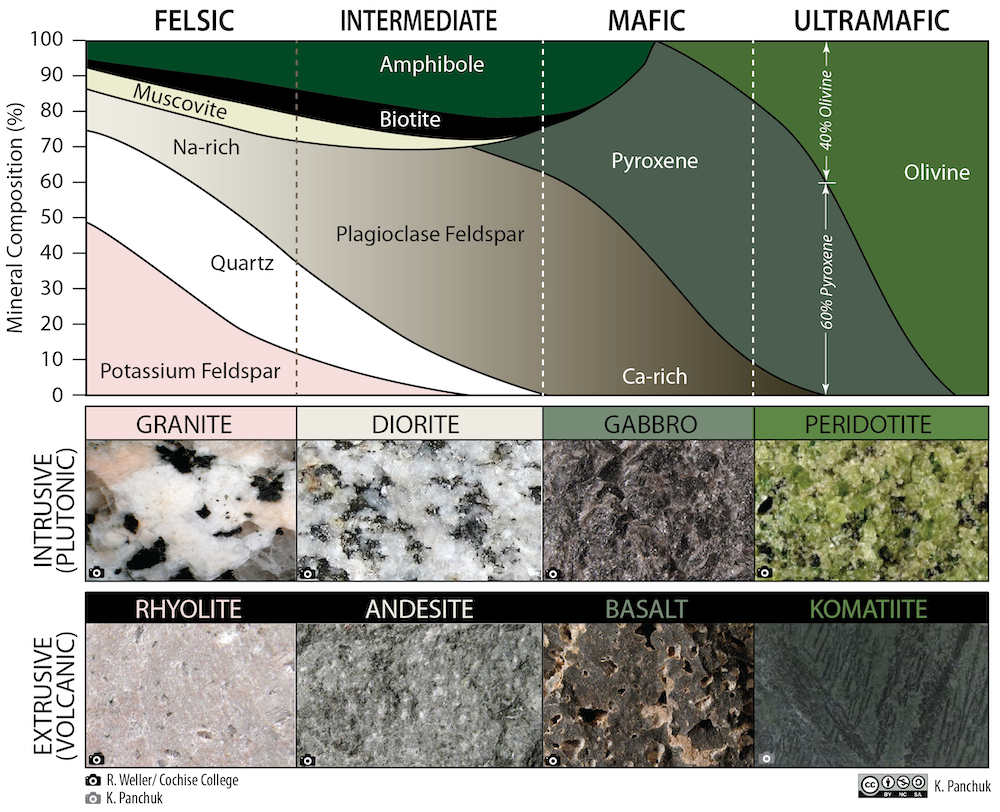
How would you identify minerals in an extrusive igneous rock if the grains are too small to see? There are a couple of tricks that geologists use to identify which type of igneous rock they have. Do you remember how color was not a diagnostic property for minerals? Well, color in an igneous rock can be diagnostic! Iron and magnesium-bearing minerals are either black or green in color, like olivine and pyroxene, and they are the primary minerals that make up mafic and ultramafic igneous rocks. Potassium, aluminum, and silica-rich minerals, like quartz and potassium feldspar, are lighter in color and make up intermediate and felsic igneous rocks. Geologists can use the color of igneous rocks and the relative amount of light- vs. dark-colored minerals to help identify them (Figures 6.2 and 6.3).
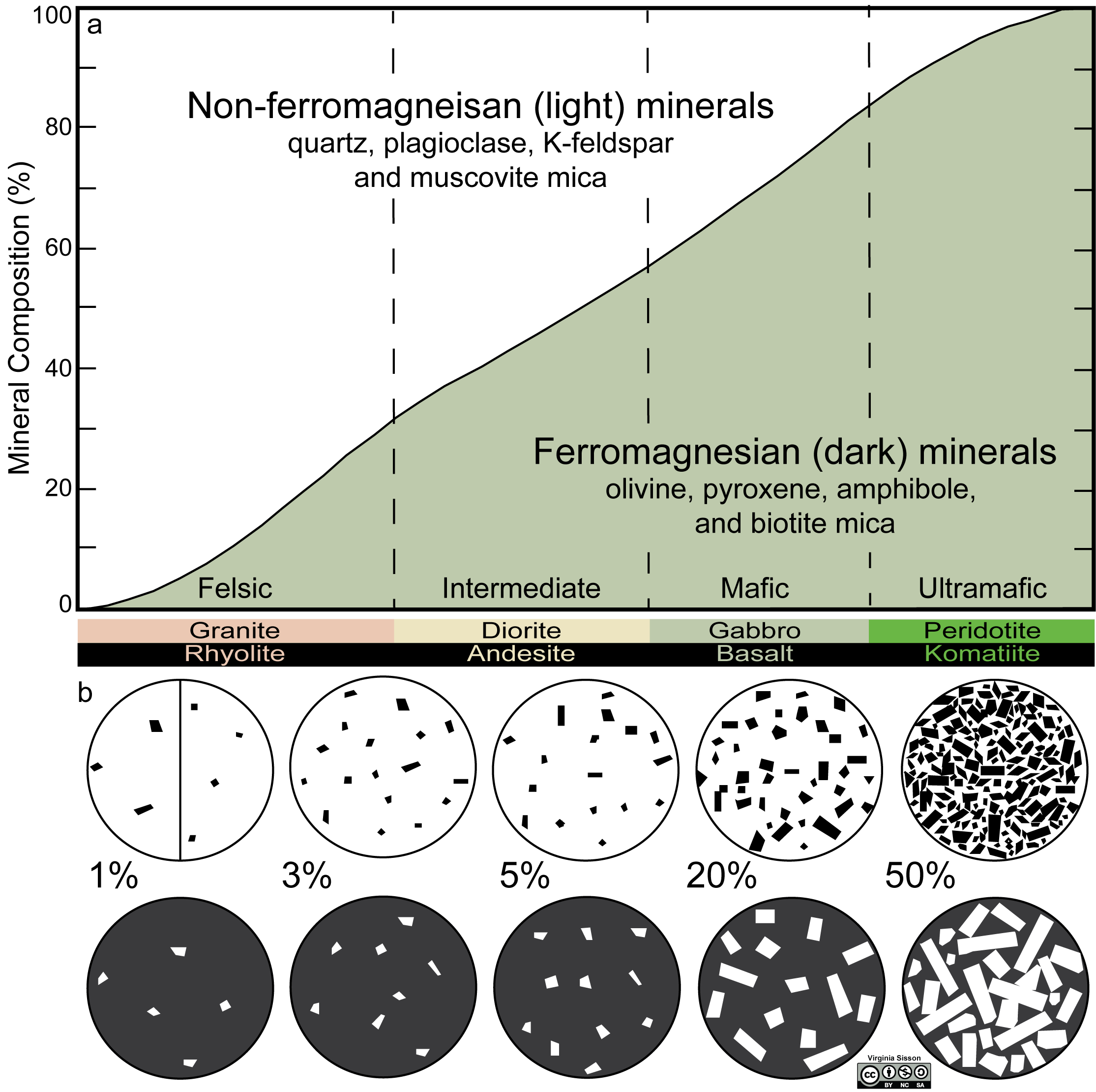
Glassy and vesicular textures are a little different (Figure 6.4). These rocks form when lava cools off too quickly for minerals to form. The conchoidal fracture in obsidian tells us that it has a high silica content (felsic) despite its dark color. The dark color comes from the tiny amount of iron present.
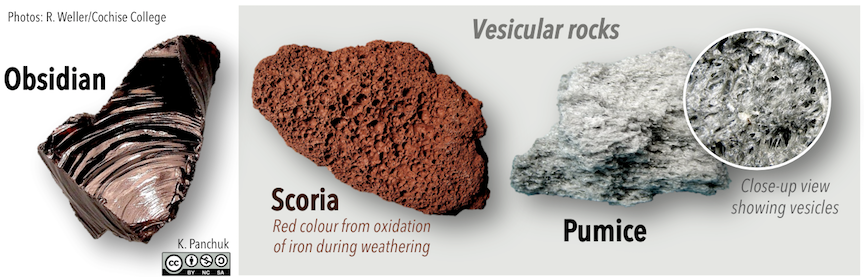
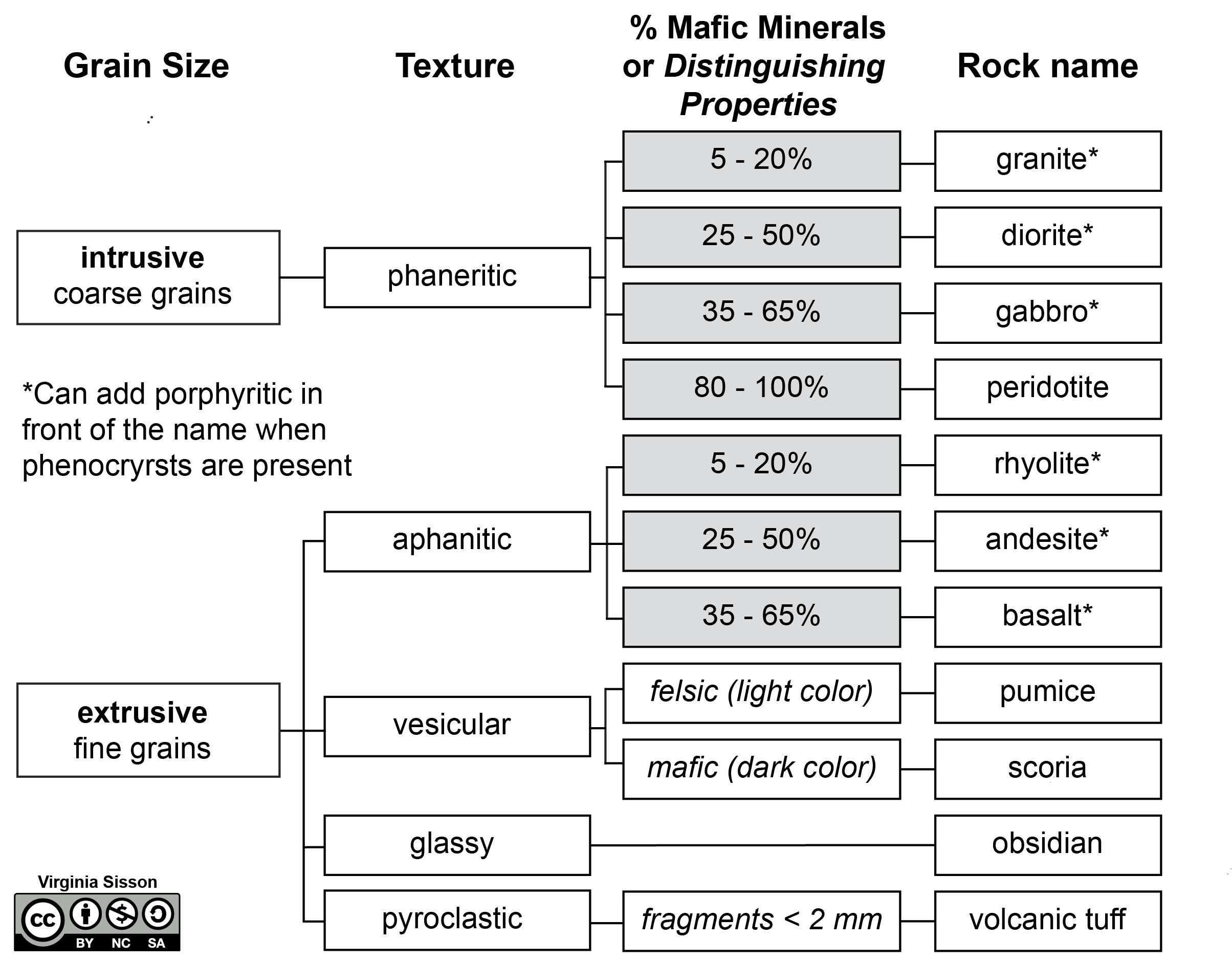
Exercise 6.2 – Igneous Rock Identification
6.3 Creating Magma
The temperatures needed to melt a rock to create magma are between 600 and 1,300 oC (1,100 and 2,400 oF), depending on the rock’s composition and its depth (pressure). With normal temperature gradients in Earth, it is almost impossible to reach these temperatures. In your lecture class, you’ll learn about decompression melting and fluid-assisted melting. It is impossible to demonstrate these in a lab setting. We can, however, simulate one melting process which results in creation of magmas.
Partial melting is when some minerals in a rock melt before others do. Most rocks are made up of different minerals, and each mineral has a different melting temperature. In general, minerals with more silica will melt before those with less silica. During partial melting, minerals with lower melting points will melt first; while minerals with higher melting points remain solid. The melt (magma) composition is “enriched” with the minerals that have melted, and the remaining solid (rock) is “depleted” in the minerals that have melted. For example, if a mafic rock undergoes partial melting, the magma created is intermediate. The resulting magma has more (enriched in) silica, and the remaining rock has less (depleted in) silica.
Exercise 6.3 – Partial Melting
Let’s construct an analog for partial melting.
- Place a scoop of cookie dough or chocolate chip ice cream into a beaker. Place the beaker on a heat source and slowly increase the temperature. What happens to the ice cream when compared to the cookie dough chunks and/or chocolate chips?
- Is your melt depleted or enriched in ice cream?
- Is your solid depleted or enriched in ice cream?
- Critical Thinking: If the chocolate chip ice cream represents a diorite, what minerals chocolate chips represent? What type of magma (melted ice cream) is generated?
6.4 Volcanoes
Volcanic eruptions are one of Earth’s most dramatic and destructive agents of change. Powerful explosive eruptions drastically alter land and water for tens of kilometers around a volcano. Also, droplets of sulfuric acid erupting into the stratosphere can temporarily change Earth’s climate. Volcanic eruptions often force people living near volcanoes to abandon their land and homes. Those living farther away are likely to avoid complete destruction, but these cities and towns, crops, industrial plants, transportation systems, and electrical grids can still be damaged by tephra, ash, lahars, and flooding.
Fortunately, volcanoes often exhibit unrest that allows eruptions to be anticipated and warnings sent to at-risk communities. The warning time preceding volcanic events typically allows sufficient time for nearby communities to implement response plans. Another way to predict future eruptions is to investigate the past eruptive history of a volcano.
Exercise 6.4 – Volcano Eruption Frequency
Some people have suggested that the number of volcanic eruptions is increasing and contributing to climate change. Let’s look at some data. Table 6.2 shows the number of volcanic eruptions worldwide from 1992 to 2008.
Year | Number of Eruptions |
1992 | 27 |
1993 | 27 |
1994 | 32 |
1995 | 33 |
1996 | 35 |
1997 | 26 |
1998 | 32 |
1999 | 39 |
2000 | 39 |
2001 | 33 |
2002 | 41 |
2003 | 29 |
2004 | 49 |
2005 | 46 |
2006 | 44 |
2007 | 36 |
2008 | 45 |
- Plot the volcanic eruption data from Table 6.2 on the blank graph in Figure 6.7.
- Do you think there is a trend in this data? Have the number of volcanic eruptions per year been increasing? Explain.
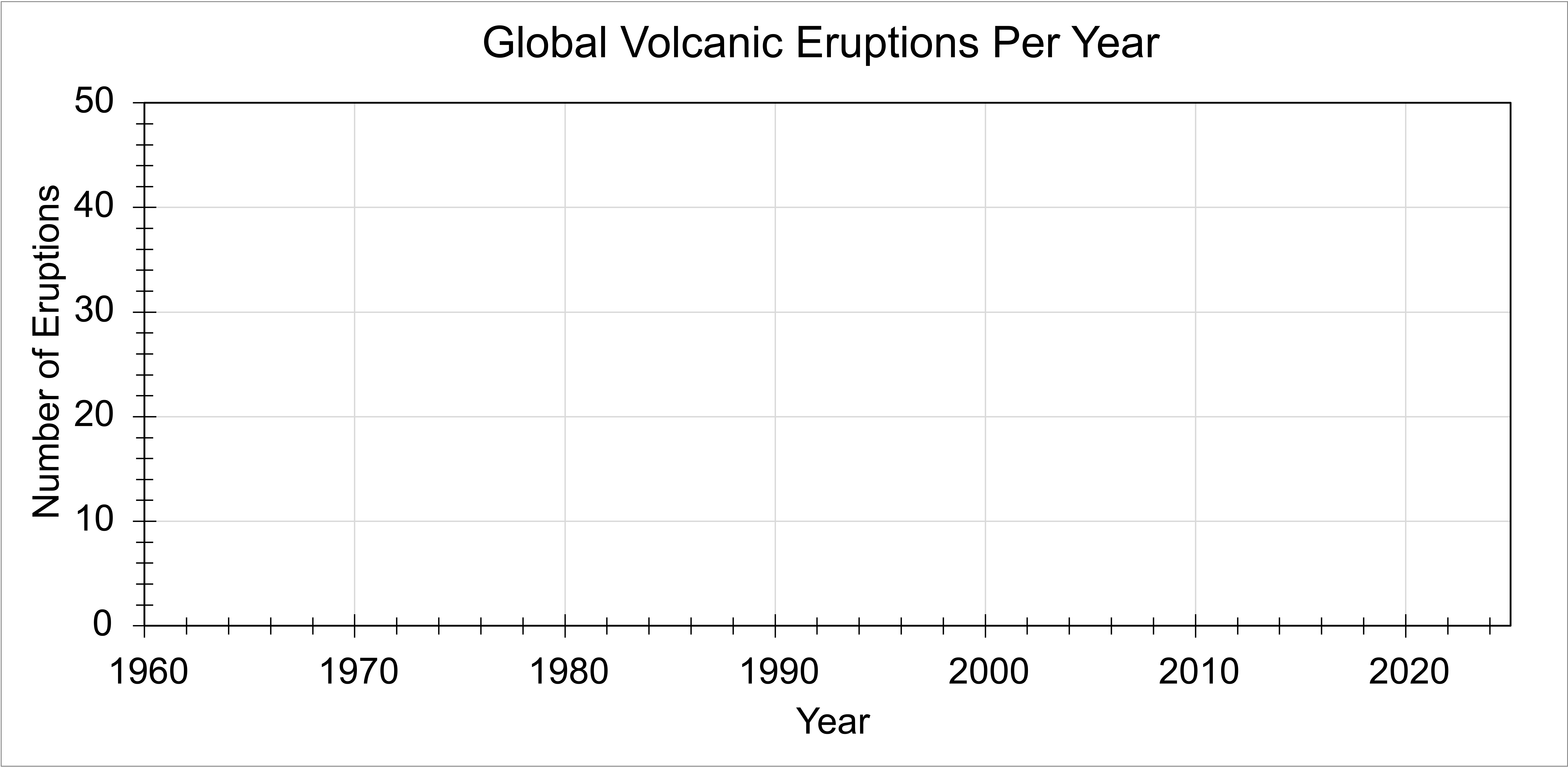
Sometimes people interpret data without looking at the big picture, either accidentally or by cherry-picking data points that support their point. You were only given a subset of the volcanic eruption data in Table 6.2.
- Table 6.3 contains the remaining data from the years before and after the data you plotted. Plot this new data on the same graph in Figure 6.7. You can use a different color if you’d like.
- Do you think there is a trend now that you have the complete data set? Have the number of volcanic eruptions been increasing, decreasing, or about the same? Explain.
Year | Number of Eruptions | Year | Number of Eruptions |
1960 | 35 | 1984 | 33 |
1961 | 32 | 1985 | 27 |
1962 | 29 | 1986 | 41 |
1963 | 40 | 1987 | 32 |
1964 | 29 | 1988 | 27 |
1965 | 34 | 1989 | 25 |
1966 | 35 | 1990 | 30 |
1967 | 43 | 1991 | 37 |
1968 | 32 | ||
1969 | 32 | ||
1970 | 34 | 2009 | 31 |
1971 | 32 | 2010 | 33 |
1972 | 30 | 2011 | 36 |
1973 | 41 | 2012 | 39 |
1974 | 27 | 2013 | 38 |
1975 | 23 | 2014 | 42 |
1976 | 29 | 2015 | 41 |
1977 | 39 | 2016 | 34 |
1978 | 25 | 2017 | 32 |
1979 | 32 | 2018 | 37 |
1980 | 41 | 2019 | 27 |
1981 | 29 | 2020 | 27 |
1982 | 32 | 2021 | 33 |
1983 | 32 | 2022 | 31 |
The volcanic eruption data is from the Smithsonian Institution Global Volcanism Program.
Geologists monitor most of the world’s volcanoes for eruptive activity, but how can past eruptions be determined, especially for events before human memories? The simplest way is to look at a detailed map around a volcano. With each eruption comes a new layer of lava that turns to igneous rock. By mapping out the igneous rocks, you can determine the volcano’s eruptive history. One of the most visited volcanoes is the Big Island of Hawaii, which is actually made up of several volcanoes, with Kīlauea being the most active today. The oldest eruption on the island started about 1 million years ago and it may be actively erupting as you read this (Figure 6.8).
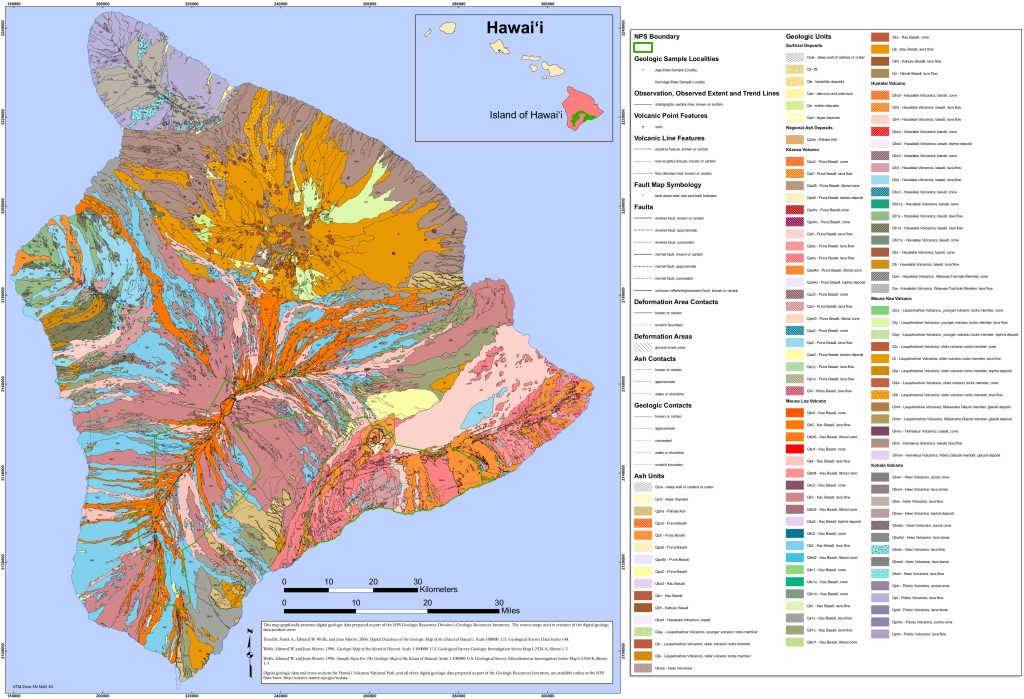
Exercise 6.5 – NASA Volcano Mapping
Volcanic mountains grow through successive eruptions of lava and other volcanic materials. Each eruption creates a new layer of rock, slowly raising the peak’s elevation. In this exercise, you’ll create your own volcano and map each eruption. This exercise is from NASA JPL. See their step-by-step instructions for images of each step.
Creating a volcano:
- Use the bottom half of a petri dish.
- Place it in the center of graph paper and trace around it with a pencil (this is your map).
- Use a paper plate and mark north, south, east, and west on its edges and then mark north on your graph paper. This will help you remember the order of events in geographic coordinates.
- Fill the petri dish with baking soda.
- Place a small amount of vinegar into the petri dish and watch the eruption of simulated lava. If it doesn’t flow, add more vinegar.
- When the lava stops, quickly draw around the flow edge with a pencil.
- Dab up the fluid with paper towels.
- Use a thin layer of play dough to cover the entire area where lava flowed.
- On one piece of graph paper, use a colored pencil that matches the play dough color to draw an outline representing the edge of the play dough, being sure to maintain the cardinal orientation (north, south, east, west) of the paper with the orientation of the volcano. Shade in this lava flow drawing. Make a note on the graph paper regarding the order of eruptions (which color came first).
- Repeat the eruption process to create a total of 4 to 6 flows.
- Trade your volcano with another group (keep your map).
Now that you have another group’s volcano, imagine yourselves as a team of geologists seeing a volcano for the first time. Your job is to investigate and map this volcano that has an unknown history.
- Grab another piece of graph paper and mark north.
- Draw the visible layer(s) of the volcano to scale on the graph paper using a ruler and colored pencils.
- How many lava flows can you see? ____________________
- Make a list of the lava flows, starting with the youngest flow at the top and the oldest at the bottom. Example: “Top flow is a long, skinny, green flow.”
- Can you easily determine the sequence of flows (which came first, second, etc.)? Are there some flows you can’t say which are younger or older? Explain.
- Are there parts of any flows that might be covered? Which ones?
- What would you need to determine the sequence and shape of each flow? How could you get that information without lifting the play dough?
Stop here and wait for your instructor before continuing.
- Complete the remaining steps outlined by your instructor. Record the locations and information gained on your map and finish it. What is the sequence of flows that tells the history of this volcano?
- Compare your map and sequence of flows with the one from the group that originally made the volcano. Was your interpretation accurate? Explain.
If you want to live near a volcano, knowing the types of hazards is critical to evaluate the risks you’ll be taking. Some volcanoes are dormant or extinct and no longer hazardous. Most countries with active volcanoes monitor their potential hazards. Active volcanoes are monitored for seismic activity (earthquakes), gases, deformation of the ground, gravity, magnetics, and thermal imaging. You can go to the Global Volcano Network to assess recent activity around the world.
Exercise 6.6 – Reading a Volcanic Hazard Map
Figure 6.9 contains a colored digital elevation model and a hazard map of the Mt. Rainier region in Washington State. For reference, Seattle is located near Puget Sound just north of this map.
- Do lava flows and pyroclastic flows pose a significant risk to the surrounding population? Explain
- What do you notice about the path lahars tend to follow in this region?
- How far can these lahars travel? Measure on the map.
- What could cause the failure of the Alder Dam and subsequent flooding (located on the western side of the map near the town of Elbe)?
- Critical Thinking: What do you think is the source of water for the lahars?

Additional Information
Exercise Contributions
Daniel Hauptvogel, Virginia Sisson, Kaitlin Thomas
size, shape, and arrangement (or fabric) of the mineral grains and crystals
Ultramafic rocks are igneous and meta-igneous rocks with a very low silica content (less than 45%), generally >18% MgO, high FeO, low potassium, and are composed of usually greater than 90% mafic minerals.
Mafic rock is silicate mineral or igneous rock rich in magnesium and iron. Mafic minerals include olivine, pyroxene, amphibole and biotite mica.
Intermediate igneous rocks are moderate amounts of silica (52-63 wt.%) and have nearly equal amounts of felsic and mafic minerals.
Felsic is an igneous rock that is rich in light-colored silicate minerals such as feldspar and quartz (silica).
a type of fracture that has a smoothly curving surface of fine-grained materials. Some say this is shell-like. This forms in material which have no planar surfaces of internal weakness or planes of separation (no cleavage). Such a curving fracture surface is characteristic of glass and other brittle materials with no crystal structure.
A volcano that is potentially active and not erupting now, but that is considered “active” because it could erupt in the future. Mount Hood is currently dormant.
volcanoes that will not erupt again