Chapter 14: Planetary Geology
Moon, Mars, and Meteorites
Learning Objectives
The goals of this chapter are to:
- Understand the evolution of rocky planets
- Compare physical features on Earth with other planets
- Apply geologic principles of Earth on other planets
14.1 Is Geology Unique to Earth?
Recent planetary missions by NASA and other space agencies have confirmed that the geological processes you have investigated on Earth also help create and shape the rocky planets and moons. Common processes link the internal structure, landscapes, and features such as volcanoes, impact craters, ice caps, dunes, faults, rivers, and oceans. Each new mission to space reveals that many supposedly dead planetary bodies still experience eruptions, landslides, and dust storms. Moreover, our understanding of the Solar System has increased by studying meteorites from asteroids and Mars and rock samples collected on the Moon.
14.2 Meteorites
At the beginning of the semester, you may have gone over the history of the solar system and how the planets were formed. Some of the evidence for what the early solar system was like and the structure of the Earth comes from meteorites because they are fragments of the early solar system. There are three types of meteorites: stony, iron, and stony-iron. So, how do you identify these? They are typically dense, possibly magnetic, have unusual shapes, sometimes with pits, and often have a “fusion crust” that forms when the meteorite falls through the atmosphere. Many possible meteorites are rocks, minerals, or slag left over from making steel.
- Stony meteorites are composed of silicates and other materials. Most stony meteorites also contain both iron and nickel metal. These are the most common type; 94% of all meteorites are stony.
- Iron meteorites are composed of iron (Fe), nickel (Ni), and sometimes cobalt (Co) and other trace elements and minerals. Iron is the predominant metal with a variable ratio of iron to nickel.
- Stony-irons are a combination of iron and stony meteorite properties and are the rarest type of meteorite. Pallasites are simply spectacular stony-irons. In a polished slice, you can see iron-nickel metal surrounding olivine crystals.
As of June 2024, 2,823 confirmed meteorites have been collected in the United States according to The Meteoritical Society, mostly in arid regions of states. In Texas, 322 have been found, and, recently, some were lucky enough to witness a meteorite fall near Brownsville, TX on Feb 12, 2023. Six days later meteorite hunters found pieces near El Sauz, TX.
Exercise 14.1 – Meteorites and Internal Structure of Rocky Planets and Planetesimals
Figure 14.1 shows three different types of meteorites. Typically, any meteorite with metal is made into a polished slab (Fig 14.1 A and D)
- Which of these is from the core of a planetesimal?
- Which represents crustal material?
- Which represents the core-mantle boundary?
- Critical Thinking: What do the materials (metal, stony-metal, and stony) imply about the early processes of planet formation in terms of planetary structure? (hint: recall that oil and water do not mix but have a meniscus between them.) Do you think that silicate and metal liquids mix or not? Explain.
Figure 14.1 Five meteorites found in Texas. A and B are iron meteorites with A as a polished slab and B unpolished piece; C is a cut slab of an aubrite; D and E are the same pallasite meteorite with D as a polished slab and E unpolished piece. Image credits: all images from Meteoritical Bulletin Database with A. Iron IIIAB found in Davis Mountains in 1903, photo by Don Edwards, ~ 1 cm across; B. Iron IIAB found in Iredell TX in 1898 photo by Don Edwards, ~1 cm across; C. Aubrite found in Pena Blanca Spring, Brewster Co TX in 1946, photo by Peter Marmet, ~3 cm across; D. Pallasite found in Sterley TX in 1950, photo by KD Meteorites, ~9 cm across; E. unpolished slab of pallasite found in Sterley TX in 1950, photo by L. Garvie, ~12 cm across.
14.3 Martian Rock Cycle
Rocky planets (or terrestrial planets) are relatively small compared to the Gas Giants. Rocky planets have solid surfaces, often pockmarked by impact craters. These don’t have rings, and they have few or no moons. They may have evidence of past or current tectonic and volcanic activity. On Earth, the rock cycle explains how the three rock types relate to each other and how they change over time. We’ve spent a lot of time talking about the rocks on Earth, but do other planets have rock cycles?
Mars, sometimes called the Red Planet, is the fourth planet from the Sun (Figure 14.2). Mars has some Earth-type characteristics, such as polar ice caps, mountains, sand dunes, and clouds. It is the planet most visited by spacecraft in our solar system. As of 2024, 21 lander missions and 8 sub-landers (rovers and penetrators) attempted to land on Mars. Of 21 landers, the Curiosity and Perseverance rovers are currently still in operation. Curiosity rover has long outlived its expected mission as it has been roaming the surface of Mars for over 11 years!

Exercise 14.2 – Martian Rock Cycle
Today, Mars is no longer volcanically or tectonically active. However, remnants of Mars’ massive volcanoes remain (Figure 14.3). Its most recent eruption is estimated to have occurred only 50,000 years ago near Elysium Mons. In fact, some attribute recent Mars quakes to movement of magmas. To distinguish features on planets from those on Earth, mountains are called Mons (singular) or Montes (plural).

- Tharsis Montes are three enormous volcanoes located within the Tharsis region of Mars. These volcanoes form a straight line each other and align with other volcanoes in the region such as Ceraunius Tholus and Uranius Mons. What does this chain of volcanoes in the Tharsis region suggest about volcanic and tectonic history of this region?
- Figure 14.4 is a picture of an igneous rock taken by NASA’s Curiosity Mars rover. The light-colored, elongated crystals are likely feldspar; some are 1 cm in size. The dark-colored matrix surrounding the feldspar is pyroxene.
- Describe the texture and cooling history of the rock.
- Is this rock felsic, intermediate, mafic, or ultramafic? How can you tell?
Figure 14.4 – Martian rock ‘Harrison’ from inside Gale crater on the lower slope of Mount Sharp. Created by merging images from two cameras on NASA’s Curiosity Mars rover. Annotated figure includes a scale bar in the upper right. Image credit, NASA, Public Domain.
- Describe the texture and cooling history of the rock.
- Figure 14.5 is a picture of a sedimentary rock taken by NASA’s Curiosity rover.
- Describe the texture of the rock.
- How much energy is needed to transport the pebbles?
- Today, the main mechanism for sediment transport on Mars is wind. What features do you see that either support or don’t support this method of sediment transport?
- If this rock were from Earth, what type of environment would you find it?
- What does this imply about Mars’s geologic history?
- At present, could there be water on Mars?
Figure 14.5 Martian outcrop ‘Link’ imaged by NASA’s Curiosity rover. Annotated with a scale bar on the lower right. Image credit: NASA JPL-Caltech/MSSS, Public Domain.
- Describe the texture of the rock.
- Do you think we could find metamorphic rocks on Mars? Use Figures 14.6 to help.
Figure 14.6 – A photo of Tooting crater on Mars. Inset shows location of crater to the west of Olympus Mons. Crater is at 23.1°N, 207.1°E, in Amazonis Planitia. This crater is estimated to be one of the youngest on Mars formed at ~1.1 Ma. Image credit: NASA, modified by Chmee2, Public Domain. - Meteorites also hold clues about metamorphism of our solar system and Mars. Figure 14.7 shows two different meteorites from Mars; one which originated from Mars during an impact about 700,000 to 1.1 million years ago and another which fell to the surface of Mars. Both of these images record possible metamorphic changes. Can you identify these processes?
Figure 14.7 – Two photos of Martian meteorites. A. Tissint meteorite that witnesses watched fall onto the Moroccan desert. B. Curiosity rover has discovered several meteorites on the surface of Mars including “Egg Rock”. Several small white pits show where the rover analyzed the rock confirming it is an iron-nickel meteorite. Also, there are abundant white veins made of calcium sulfate (anhydrite, bassanite, and perhaps gypsum). Image credit: A: James St. John, CC BY; B: NASA, Public Domain. - Another way to investigate planetary surfaces is to use satellites equipped with spectrometers such as CRISM (Compact Reconnaissance Imaging Spectrometer for Mars). CRISM uses detectors that see in visible, infrared and near-infrared wavelengths to map the minerals that have water in their structure. This spectrometer surveyed the surface of Mars from March 2006 until January 2023. Figure 14.8 shows a geologic map of the Terra Sirenum region with relative ages based on impact relationships. Also shown are some of the CRISM results showing mineral locations for both minerals in igneous rocks (primary minerals) as well as secondary minerals formed by either metamorphism, hydrothermal activity or evaporite (lake systems).
- Look at the distribution of primary minerals. Do they occur only in impact craters? What is their age?
- Look at the distribution of secondary minerals. Do they occur only in impact craters? What is their age?
- The presence of gypsum (a common variety of polyhydrated sulfate) may indicate evaporite deposits. Do these occur only in impact craters? What is their age?
- Critical thinking: According to Viviano et al. (2023), this region has 30% more secondary minerals than other areas on Mars. Why do you think this area has more secondary minerals?
- Look at the distribution of primary minerals. Do they occur only in impact craters? What is their age?
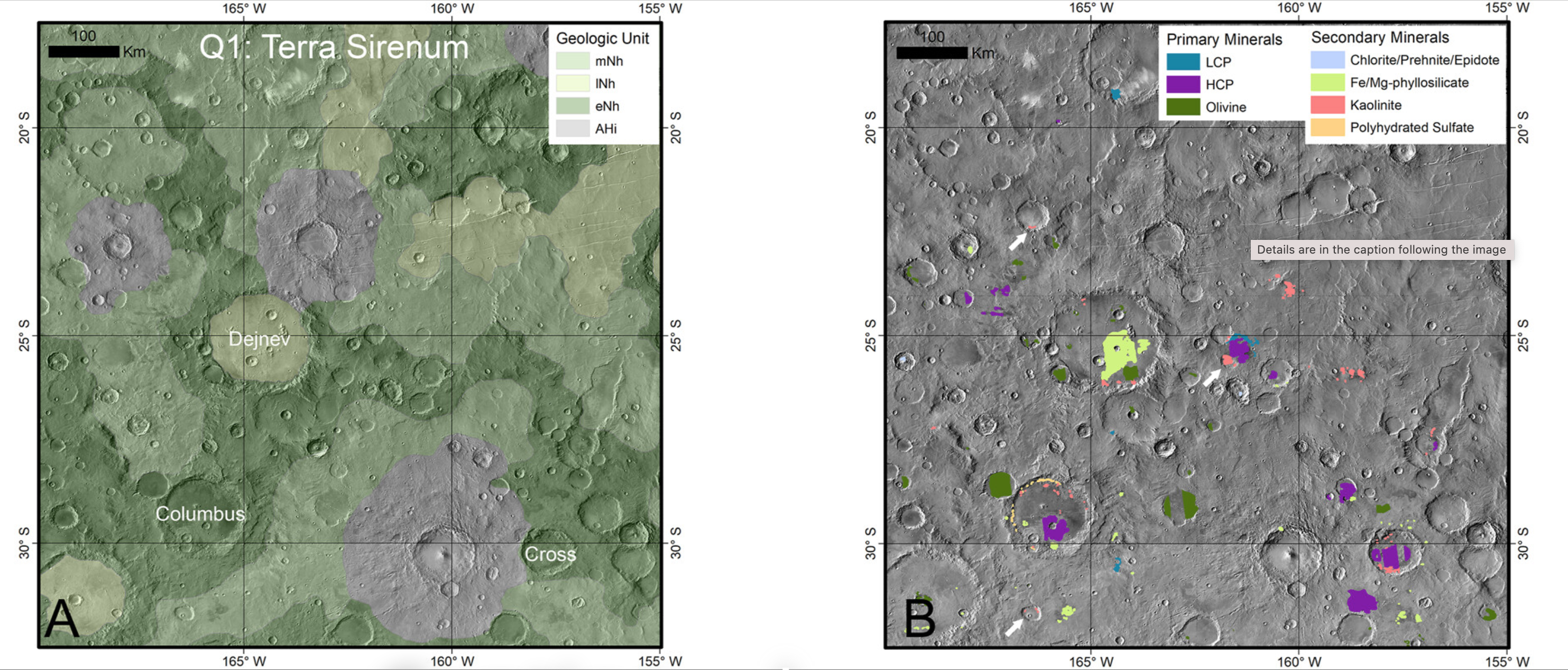
Exercise 14.3 – Lunar Geology
Other planets and moons have shown evidence of geologic activity, like Earth’s moon. Head to this interactive map of the Moon created from images with the Lunar Reconnaissance Orbiter Camera.
- Separate the moon’s surface into different types using any criteria. What are the criteria you used and why?
- Share your criteria with the class and compare answers.
Hopefully, you picked up on the distinction between two different colors on the Moon’s surface: a lighter shade of white or gray and darker colored areas. The lighter areas are the lunar highlands and are composed of anorthosite, a special type of granite with mostly calcium-rich feldspar. The darker areas are the lunar mare or maria, composed of a special type of basalt called KREEP, which has high concentrations of potassium (K), rare-earth elements (REE), and phosphorus (P).
- Describe the distribution of craters on the moon. Is there any distinction between the highlands and the maria?
- You may have heard about recent attempts to land on the South Pole of the moon with two failed missions from Japan and Russia and two successful missions including India’s Chandrayaan-3 and the short-lived Odysseus mission by Houston based Intuitive Machines . NASA has proposed that the first Artemis III flight land in this region in 2026. Describe the landscape in this region. Why is there renewed interest in landing in this region?
- Critical Thinking: Some areas of the moon have more craters than others. Why? Note: Under the layers tab > ACT Layers, turn on TerrainHillshade to really see the difference.
14.4 Relative Dating in the Solar System
There are thousands of craters on the moon, Mars, and other planets, and only ~190 on Earth. Planetary scientists use the law of superposition with these craters to determine the relative ages of the moon and other planets. As you found out in Chapter 10 on geologic time, cross-cutting relationships is a principle in geology used to decipher the relative ages of rocks and geologic features. This principle is based on the concept that a rock or feature must be younger than any rock or feature it cuts across. We can apply this principle to determine the relative ages of features on other planetary bodies. For example, the surface of Mercury has many landforms, including ridges (rupes) and craters. Figure 14.7 shows that the ridges may be younger than some craters yet older than others. Some craters even cross-cut other craters!

Exercise 14.4 – Relative Dating on Mars
Figure 14.10 is a Google Earth image from Mars that shows craters, fractures, and river channels. Use the principle of cross-cutting relationships to answer the following questions.
- Which is older, Crater A or Fracture A? How can you tell?
- Which is older, Crater A or Channel A? How can you tell?
- Is Crater B younger or older than Crater A? How can you tell?
- If Fracture B cuts through Channel A, is Crater A younger or older than Crater C?
- Look at Figure 14.11. Arrange five of these craters in relative order from oldest to youngest.
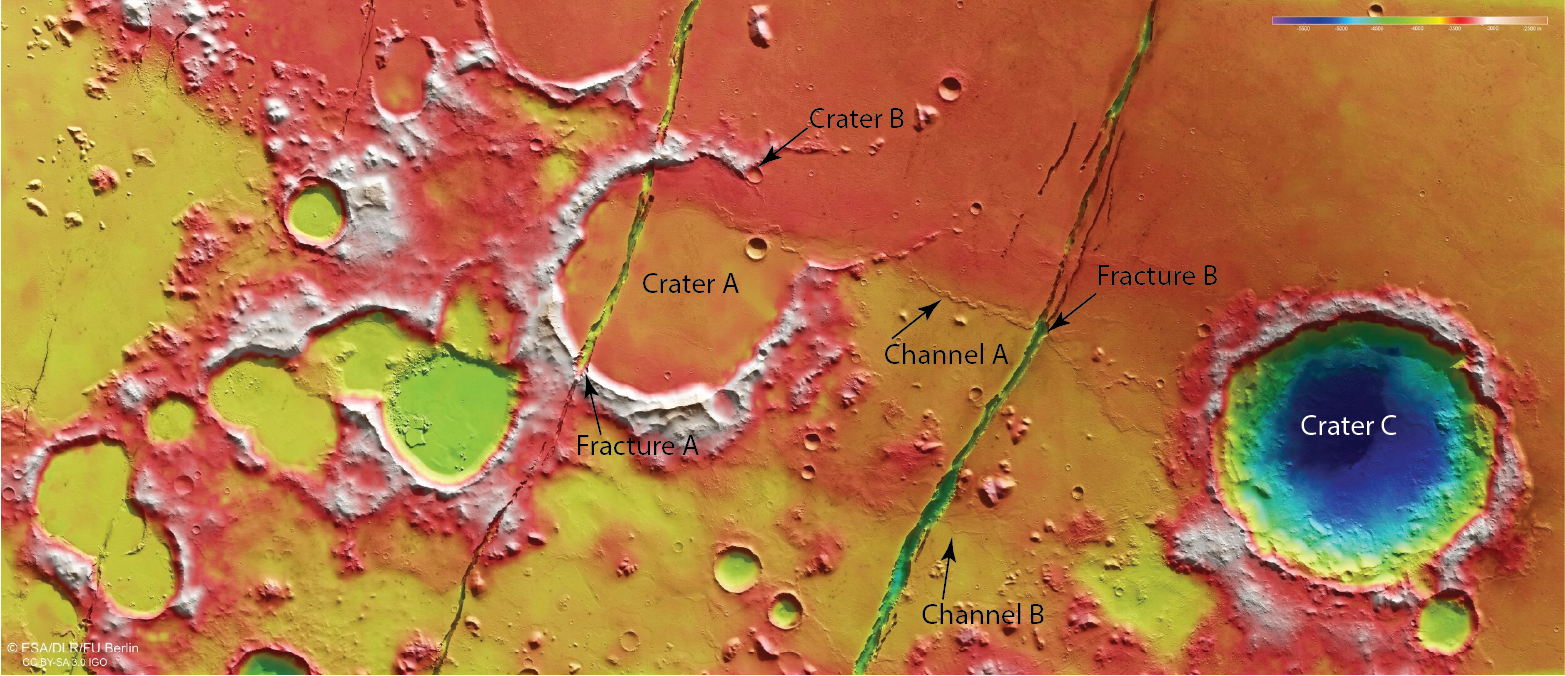
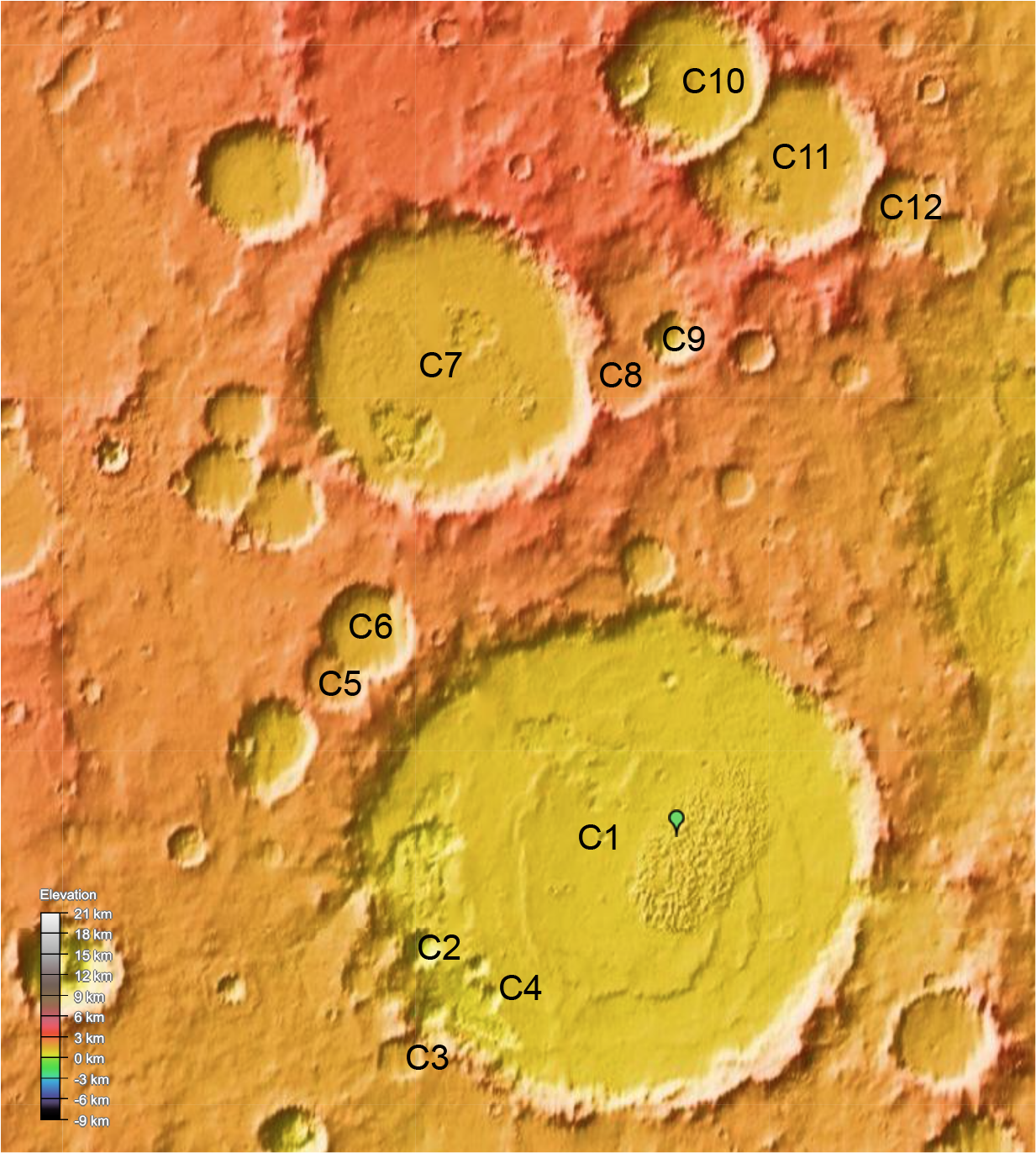
14.5 Planetary Dunes
As you learned in the landscape chapter, dunes are classified by the amount of sand and wind direction. On Earth, vegetation also influences dune morphology, but vegetation is not an issue in the rest of the solar system. The sand that makes up planetary dunes is just small solid particles, which can be basaltic or even solid methane if you are in the outer solar system. Planetary geologists have identified dunes on Earth, Venus, Mars, Titan (moon of Saturn), Triton (moon of Neptune), Io (moon of Jupiter), Pluto, and at least one comet.
Exercise 14.5 – Winds and Dunes on Mars
In this exercise, you’ll look at some images of dunes on Mars (Figure 14.2). To interpret these, look for shadows on the dunes. This is the steep versus gentle slope on the dune. The steep side will be the downwind side for barchan, transverse, barchanoid, and star dunes. When looking at these images, you must ignore the features that are not dunes, such as hills, mesas, buttes, craters, etc.
- Figure 14.12 shows several dunes from Mars. On each image, use arrows to show the direction(s) of the wind. Also mark a spot where you might land to explore this region.
- For each image, determine the dune type, number of wind directions, and relative amount of sediment. Fill in this information in Table 14.1.
Table 14.1 – Martian Dune Data Location Dune type(s) # of wind directions Sediment amount A. Summer dunes (43° N) B. Dunes in crater (12° S) C. Winter Dunes (76° N) D. Chasma Boreale (85° N) Figure 14.2 – Four false-color images of Martian dunes. A. Dunes sitting on white ridges of gypsum that are deposited on basalt. B. Dunes formed within an impact crater with mound in the center that appears to have blocked the wind. C. Dunes with frost. Image collected on Martian winter solstice (7/22/22). This frost is mixture of carbon dioxide and water ice. D. Dunes on Mars from the north polar ice cap in the Chasma Boreale region. The white areas show irregular polygonal patterns developed in carbon dioxide ice (dry ice). The dunes are made of basalt and gypsum grains. Image credit: False-color HiRISE image from NASA/JPL-Caltech/UArizona Public domain. - Now that you’ve analyzed dunes from several places on Mars, let’s see if you make any generalizations about winds and sediment supply on the Mars. Do these all places have only one direction of wind.
- Does the number of wind directions change with distance from the Martian equator?
- Is the sediment supply the same in the different Martian examples? Rank them from most to least amount of sediment.
- Critical Thinking: Which of these dunes fields is where you would like to go explore? Just choose one and explain why you chose that place.
Additional Information
Exercise Contributions
Virgina Sisson, Kaitlin Thomas, and Daniel Hauptvogel
References
Hare, T.M, R.K. Hayward, J.S. Blue, and B.A. Archinal, 2015, Image mosaic and topographic map of the moon, 2 sheets, U.S. Geological Survey Scientific Investigations Map 3316, doi:10.3233/sim3316
Tanaka, K.L., Robbins, S.J., Fortezzo, C.M., Skinner, J.A., Jr., and Hare, T.M., 2014, The digital global geologic map of Mars: Chronostratigraphic ages, topographic and crater morphologic characteristics, and updated resurfacing history, Planetary and Space Science, v. 94, p. 11-24., doi:10.1016/j.pss.2013.03.006
Viviano, C.E., Beck, A.W., Murchie, S.L., Dapremont, A.M., and Seelos, F.P., 2023, Heterogeneity of the Noachian crust of Mars Using CRISM multispectral mapping data, Geophysical Research Letters Volume 50, Issue 5 e2022GL102711: doi:10.1029/2022GL102711